Blinded by the light: Starshades will dim starlight so space telescopes can get a better look at exoplanets
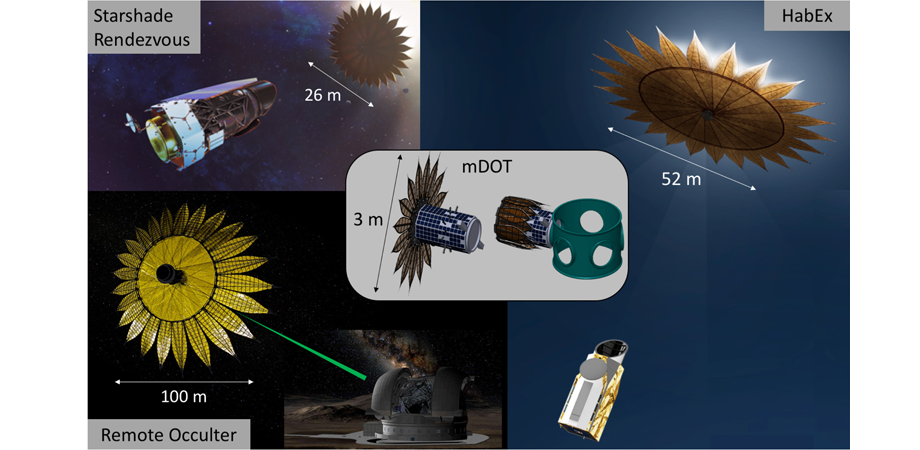
Since 1989, NASA has confirmed the existence of 4,401 exoplanets—planets outside our solar system—and 3,271 stars with confirmed planets orbiting them. Scientists’ efforts are now being directed to getting a better look at exoplanets to assess their capacity to support life, but the stars they orbit blind telescopes with their light.
That’s where starshades come in. Sampling light from an exoplanet separately from the light of its host star enhances the ability to measure the planet’s size, orbit, and other metrics that tell us about its potential habitability and possibly of the presence of life. However, the faintness of exoplanets in relation to their brighter host stars makes observing them difficult. To suppress the starlight, starshades—external light shields onboard space telescopes—can be used so exoplanets can be more easily observed and measured.
Starshades extend capabilities toward directly imaging Earth-like exoplanets—planets orbiting their stars within a zone in which water can exist on the planet surface—and toward exploring the atmospheres and terrain of the exoplanets.
A recently published collection of papers on starshades in the Journal of Astronomical Telescopes, Instruments, and Systems examines recent advances in this rapidly developing technology that has attracted the interest of many institutions. The 19 articles provide an overview of the technology with topics including starshade programs and missions, formation flying, deployment, high-contrast imaging, and performance modeling.
Guest edited by Jonathan W. Arenberg of Northrop Grumman Space Systems, Anthony D. Harness of Princeton University, and Rebecca M. Jensen-Clem of the University of California, the special section serves as a tutorial for potential investigators not yet familiar with starshades.
In an editorial, the guest editors provide a fictional dialogue between a hypothetical student, Morgan, and a starshade expert, Urania. Together, they discuss everything you want to know about starshades. The excerpt below covers the scientific reason behind the flower-shaped starshade design.
Morgan: Let’s start with the simplest question there is: what is a starshade and how does it work?
Urania: Let’s take a look at the image below, which shows the architecture of a starshade. Morgan, please note that this picture is not at all to scale but is drawn this way for its pedagogical purposes. From left to right, there is the host star and its planet. The starshade is next: it is a shaped optic of radius R, typically tens of meters. We also call it a binary optic, meaning the transmission is either complete, unity or 1, or entirely opaque, null or 0. The starshade resides on the line connecting the telescope at right and the target star at left. The spacing between the starshade and the telescope is typically tens of thousands of kilometers and is called Z. As shown in the picture, the starshade casts a dark shadow and the telescope sits in it. This shadow effectively turns “off” the host star. The light from the target planet, which is off-axis relative to the star, is not attenuated and is collected by the telescope.
Illustration of the starshade architecture. A starshade of radius R located at a distance Z from a telescope creates a dark shadow, which suppresses the starlight while allowing the faint exoplanet light to enter the telescope unimpeded. Credit: Arenberg et al.
Morgan: So, the telescope is looking over the edge of the shade, sort of like a total solar eclipse. Is it really that simple?
Urania: Yes, conceptually it is. Implementation is a bit harder, though. In fact, if you look carefully in the literature, you can see that external occulters have been around since the 1940s for use in solar astronomy. Apodized or “toothed” apertures enter that literature in the early 1960s.
Morgan: Speaking of “toothed” apertures, why are starshades shaped like flowers?
Urania: Starshades are shaped like flowers to control the diffraction of starlight around them and create a dark shadow in the image formed by the telescope. To begin to understand this fundamental aspect, let’s consider the next image, which shows a simple circular disk as the black region. You also see what are called Fresnel zones, alternating gray to white, which are a mathematical construct essentially counting the difference in path length … between the on-axis point and the radius of the zone. This difference in path length causes phase changes that determine how the electric field adds up.
Circular disk, with Fresnel number of 14, in front of alternating gray and white Fresnel zones (shown out to the 26th zone) Credit: Arenberg et al.
Morgan: It looks to me like the distance is the same for the entire edge of the circle. Light diffracted from the edge of the circle travels the same distance?
Urania: Yes, exactly.
Morgan: So everything has the same phase and adds up to the bright on-axis spot.
Urania: Yes, Morgan, you got it!
Morgan: So that is how we get the Spot of Arago! For any circle all the distances and phases add up constructively.
Urania: Correct. Since we want to “turn off the star”, we want destructive interference, so the diffracting screen needs to vary the distance and therefore the phase. … In early literature this was called a toothed aperture. Toothed apertures are well known to suppress diffraction effects.
Morgan: Let’s see if I understand this critical piece. Our goal is to get all the light that diffracts from the starshade to the entrance aperture of the telescope to be a dark null. This will happen when light with positive phase exactly cancels the light with the opposite phase—total destructive interference.
Urania: Exactly! Here’s another way to understand how it works. We can think of the starshade as consisting of two components: (1) a central circular disk and (2) radially tapered petals attached to the disk. The central disk serves as the occulting spot that blocks the on-axis source and creates a geometric shadow that covers the telescope. However, as we just discussed, light will diffract around the sharp transition of the disk’s edge, spoiling the darkness of the shadow. This is where the petals come in: to create a smooth reduction in the transmission of the disk to reduce the diffractive ringing caused by the sharp truncation of the electromagnetic field. This process is called apodization and is a common problem in optical design and engineering.
Learn more about starshades in the full dialogue in the guest editorial: Jonathan W. Arenberg, Anthony D. Harness, and Rebecca M. Jensen-Clem, “Special Section on Starshades: Overview and a Dialogue,” J. Astron. Telesc. Instrum. Syst. 7(2), 021201 (2021) doi: 10.1117/1.JATIS.7.2.021201
Enjoy this article? Get similar news in your inbox |
|