How to build an optical quantum computer
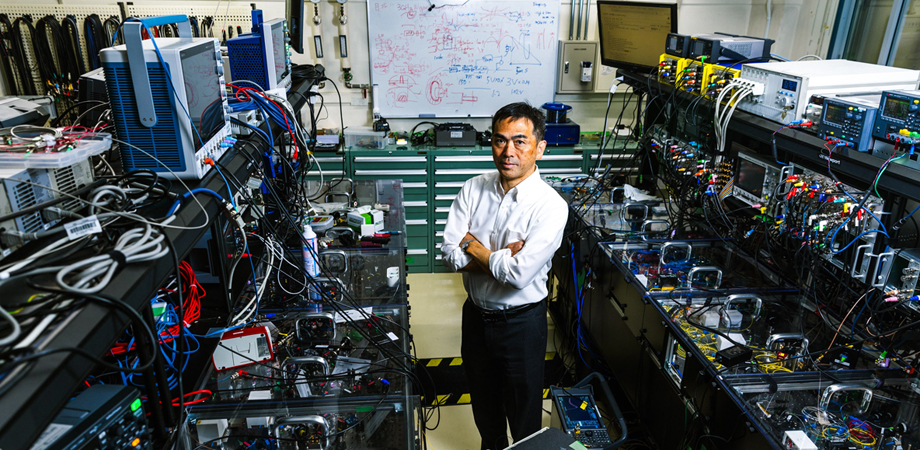
Back in 1998, an international team of physicists including Akira Furusawa teleported a quantum state of light across one meter, without it travelling through any kind of physical medium. Furusawa, working with Jeff Kimble at Caltech’s quantum optics lab, and colleagues from the Universities of Aarhus in Denmark and Bangor, Wales, used a bunch of optics, receivers, and control systems to create quantum entanglement from two squeezed states of light. They then ‘transported’ these beams of entangled photons from one side of their optical bench to the other. Critically, the team had disassembled and then reconstructed this quantum state with such high fidelity that the community at large agreed this to be the first ‘true’ teleportation of a quantum state. Results were published in Science.
“Our key tools were low-loss optical parametric oscillators, high-quantum-efficiency homodyne receivers, and optical phase control systems,” Furusawa tells Show Daily. “With these we created Einstein-Podolsky-Rosen-type of entanglement [and other necessary steps] to realize quantum teleportation — it was awesome.”
And indeed it was. Before this time only simpler quantum systems, such as a photon’s polarization, had been teleported. But by achieving this feat with a stream of entangled photons, Furusawa and fellow physicists had flung open the door to quantum computers, scalable quantum networks and a quantum internet.
Post-teleportation breakthrough, Furusawa returned to Japan, joined the University of Tokyo, and continued to work on quantum teleportation with the lofty goal of eventually building an optical super-quantum computer. Since this time, he and colleagues have been painstakingly constructing the necessary technology to get there — they’ve nearly made it, and here’s how.
Step by step
Six years after his first teleportation breakthrough, Furusawa extended two-way quantum teleportation by building a three-way experimental network based on the quantum entanglement of electromagnetic states. Then, by 2009 he had developed a more complex teleportation network of nine entangled optical beams, and was heading towards the large and critical ‘continuous-variable cluster state’ over which computations can take place. “We’d realized quantum error correction for continuous variables,” he highlights.
Furusawa’s Tokyo University team then joined forces with Elanor Huntington and colleagues from the University of New South Wales, Australia, and made worldwide headlines in 2011 when they teleported wave packets of light — across bandwidths up to 10 MHz — in a Schrödinger’s cat state. Key experimental steps were to construct broadband, zero-dispersion apparatus that could work with time-resolved equipment to deconstruct light wave packets into a continuous stream of infinitely small time bins for teleportation at high bandwidths. As Furusawa recalls: “We’d succeeded in broadening the bandwidth of our quantum teleportation systems — and in particular had created broadband squeezed light.”
Within a few years, Furusawa and colleagues had honed their time-resolved broadband teleporter set-up to efficiently transfer several entangled photonic qubits. Then, working with Nicolas Menicucci, now at RMIT University, Melbourne, Australia, the Tokyo University team swiftly scaled-up entanglement by multiplexing light in the time domain, generating massive continuous-variable cluster states containing more than 10,000 entangled wave packets of light. Teleportation-based quantum computing was tantalizingly close.
As Furusawa, Menicucci and colleagues wrote in their 2013 Nature Photonics paper, ‘Ultra-large-scale continuous-variable cluster states multiplexed in the time domain’: “The time-domain multiplexing approach allows each additional quantum mode [individual wave packets of light in two beams] to be manipulated by the same optical components at different times, which is a powerful concept.”
But while the team’s 10,000-plus entangled wave packets had formed by far the largest entangled-state ever created — at the time — a mighty one-million-mode continuous-variable cluster state was achieved in 2016, with a large-scale universal continuous-variable cluster state following in 2019. In this latest experimental set-up, laser beams were directed onto four optical parametric oscillators to produce squeezed light states, which were then woven together by beamsplitters and optical delay lines into the gigantic entangled continuous-variable cluster state.
Size is everything in Furusawa’s measurement-based quantum computing as the number of entangled wave packets of light, and their entanglement, determines the resource space available for calculations. Researchers’ analyses indicated that their cluster state was certainly entangled enough for quantum computation and they were edging ever-closer to the much-coveted ‘fault-tolerant’ computing that avoids the cascade of errors that can thwart quantum calculations.
Lighting up quantum processing
Continuous-variable cluster states are not the only route to quantum computing. For years, quantum computing mostly relied on either superconductor circuits or trapped ions to create qubits — the all-important units of quantum information. Superconducting qubits are under development by the likes of IBM, Google, and Canada-based D-Wave while Honeywell, IonQ, and others are pursuing the trapped-ion approach. For each set-up to function and fault-tolerant computing to be achieved, the qubits must be isolated from environmental noise, under cryogenic or vacuum conditions, to reduce errors and prevent the quantum state from collapsing.
Optical approaches are different, and can be run closer to room temperature. For example, PSIQuantum, has been working with GlobalFoundries on single-photon qubits, based on silicon photonic chips. The California start-up recently won funding from DARPA to develop a million-qubit processor for fault-tolerant computing, and a first commercial quantum computer is expected in the next six years.
A superconducting nanostrip photon detector developed by Akira Furusawa and colleagues as part of their experiments to generate Schrödinger cat states in 2022. Credit: Kan Takase, University of Tokyo.
Likewise, continuous-variable cluster state quantum computing, as pursued by researchers, such as Furusawa, and start-ups, including Xanadu of Canada, is amenable to room temperature operation. However, proponents also believe these cluster states, which are used for quantum calculations instead of photon qubits, are easier to produce at scale compared to the single photon set-up. Indeed, for Furusawa and colleagues, progress has been undeniably swift and ambitions are growing — as Furusawa described in his Plenary Presentation, ‘Optical quantum computers with quantum teleportation’, on Monday at Photonics West..
In short, the researchers now intend to combine their optical quantum teleportation breakthroughs with wireless network technologies, to build a ‘real machine’ of optical quantum computers. Results from just last year, revealed how Furusawa and colleagues from NTT Device Technology labs and the RIKEN Center for Quantum Computing, exploited their time-domain multiplexing approach to quantum entanglement, to get ever-closer to large-scale and high-speed quantum processing for fault-tolerant computing. The researchers combined a broadband balanced photodiode, typically used in commercial coherent wavelength-division multiplexing (WDM) telecommunications in 5G networks, with an optical parametric amplifier to squeeze and entangle broadband light enough to generate a cluster-state for quantum calculations.
‘A kind of quantum teleportation’
In his plenary, Furusawa highlighted how next-generation telecom tech — including 6G networking components — can be harnessed to deliver a powerful, ultrafast, multi-core optical quantum processor — which is what he is building right now. The quantum computer set-up will include waveguide optical parametric amplifiers to create 10 THz bandwidth squeezed light, 100GHz-bandwidth 5G/6G technologies and WDM, and non-linear feed-forward. The aim is to achieve, as Furusawa says, ‘a kind of quantum teleportation with 10 THz bandwidth this year’, and then work on the 100 GHz clock, 100-multicore super quantum computer. “The computer will work at room temperature and we should realize it within the next ten years,” says Furusawa. “It should be faster than a conventional computer that has a several GHz clock frequency.”
The waveguide optical parametric amplifier (OPA) module developed by Akira Furusawa and colleagues — they combined this with a photon detector to squeeze and entangle broadband light as part of their experiments to generate Schrödinger cat states in 2022. Credit: Kan Takase, University of Tokyo.
To commercialize this technology, Furusawa also intends to launch a start-up later this year that will eventually provide large-scale ultra-fast fault-tolerant optical quantum computers. But first, the new company will develop quantum computers for running quantum neural networks. These powerful networks hold great potential for certain types of big data analysis, and have already demonstrated that they can have a higher capacity and describe more functions than traditional neural networks. Depending on the architecture, the neural networks can also be trained more quickly.
“This year, we will make a real machine [based on an] optical quantum computer with a modest speed, such as 100 MHz clock frequency, that enables a neural network,” says Furusawa. “It should be very efficient, and moreover, when we also use 5G/6G telecommunications technologies, the clock frequency can reach 100 GHz.”
Indeed, Furusawa is eager to convey the value of next generation telecoms tech in quantum computing, as part of his Plenary Presentation. “The most important message in my talk is that importing the excellent 5G/6G technologies to optical quantum computers can realize ultra-fast optical quantum computers,” he says. “The goal of our start-up should be to provide these large-scale ultra-fast fault-tolerant optical quantum computers — but progress needs to be step by step.”
This article was originally published in the Photonics West Show Daily in January 2024.
Enjoy this article? Get similar news in your inbox |
|