The mantis shrimp: From ocean predator to optical inspiration
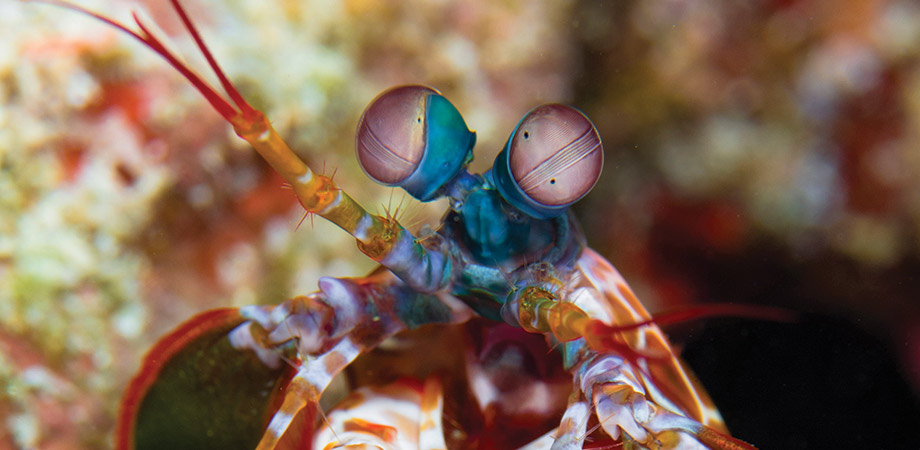
“When I was a grad student, we went out on boat trips and we would just bring up animals to see what we got, and on one patrol I saw a mantis shrimp,” recalls marine biologist Tom Cronin of the University of Maryland. “I had no idea what this thing was—it was fairly large, in this case about six inches long, and had big green eyes—and I thought, “There must be something known about this crazy animal.”
In fact, very little was known about mantis shrimp at the time. More than 40 years later, the situation has changed somewhat, but Cronin remains utterly fascinated by these unique crustaceans. “They’re like the goose with the golden egg: every new fact you unearth about them is pretty much a mantis shrimp specialty.”
Two mantis shrimp specialties outstrip the best that the rest of nature (and science for that matter) can muster. The first is an incredibly strong and lightweight club some species use to smash their prey. The second is the most complex visual system ever observed in the animal kingdom.
Their two eyes are a natural marvel. Mounted on stalks, they move independently, continually scanning their environment. Each eye is compound, with two hemispheres separated by a thin midband running between them. Zooming in, the eyes consist of tens of thousands of ommatidia. An ommatidium has a cornea that focuses light onto a series of photosensitive cells that can perform different functions.
In the eye’s two hemispheres, ommatidia collect depth, linear polarization, and light intensity information, while in the skinny midband, they sample color and circular polarization information. The mantis shrimp’s brain then has the unenviable task of simultaneously interpreting this cornucopia of visual input.
What this means is that the world that mantis shrimp perceive is far richer than humans or any other animal could possibly imagine. Mantis shrimp possess 12 color- channels, dwarfing the three (red, blue, green) found in humans, allowing them to sample wavelengths beyond the visible, ranging from ultraviolet (UV) to infrared (IR). What is more, they can sense polarized light—much like polarized sunglasses—either extracting the light whose electric field travels along a single plane (linearly polarized) or on a clockwise or counterclockwise rotating plane (circularly or elliptically polarized). In fact, mantis shrimp are the only creature known to be able to sense circularly polarized light.
A close-up of the unique architecture of the mantis shrimp eye. Photo credit: Roy L. Caldwell/University of California, Berkeley.
Good color vision no doubt contributes to the mantis shrimp’s ability to find mates, navigate, and hunt prey in coral reefs—the spectrally rich environment that they call home. And polarizing reflections are known to help crustaceans identify objects and enhance contrast, with the bodies of some (including mantis shrimp) sporting polarization patterns as signals to potential mates and other species.
Yet many questions remain: Why they need so many color channels, why their color vision is restricted to a thin midband region of their eyes, and why they evolved to detect circularly polarized light are just a few oddities about how the mantis shrimp perceive the world that remain mysterious.
However, these mysteries have not prevented technology innovators drawing inspiration from the mantis shrimp’s unique visual system. One of the first to do so was Viktor Gruev at the University of Illinois at Urbana-Champaign. He came across the mantis shrimp’s unusual visual traits almost by accident. Gruev had spent eight years developing nanoscale wires that polarized light just as it reached a CCD sensor. In 2010, he published results from his device, the first polarization camera in the visible spectrum.
Although he built the camera, Gruev did not know what to do with his invention. Fortunately, Cronin—having read Gruev’s paper about the camera—did. The pair began collaborating, with Cronin and his team using and customizing Gruev’s camera as a tool to study mantis shrimp off the coast of Australia. In turn, Gruev’s group began to learn a lot about mantis shrimp, particularly their visual system, from Cronin.
“I think working with a marine biologist changed my perception of how we can build sensors,” says Gruev. He found out that the mantis shrimp visual system uses vertically stacked photodetectors, where in one photosensitive column there are multiple photosensitive elements tuned to different wavelengths. Deeper and larger photosensitive cells are most sensitive to longer wavelengths, while shallower and shorter photosensitive cells are sensitive to shorter wavelengths.
“I came to realize that these creatures are actually using multiple photoelements in a very clever, space-efficient, information-efficient manner,” says Gruev. “We started thinking how to design a vertically stacked camera with polarization gratings that really mimics the color polarization of the visual system of the mantis shrimp—that’s where our bioinspired journey began.”
With potential end uses in mind that included helping photographers discern details even in bright light and assisting autonomous cars in spotting hazards through shadows and fog, Gruev upgraded his device from a grayscale to a color polarization camera. “When we developed the first bioinspired color polarization sensor, this was one of those proud moments because it was groundbreaking,” says Gruev.
Since then, a clutch of other innovators has discovered the mantis shrimp, and derived inspiration from its unique vision. For instance, around 2016, after having read an article about mantis shrimp, Arizona State University’s Yu Yao began thinking about how to mimic the mantis shrimp’s ability to sense circularly polarized light in the development of an advanced microscope.
Image contrast in conventional optical microscopes mainly comes from differences in light intensity and color, but Yao wanted to engineer a microscope with superior contrast based on how objects interact with polarized light. Such devices existed but were bulky, slow, and expensive. Yao’s approach to overcoming these barriers was to mimic the layered structure in the eye of the mantis shrimp but with compact and cutting-edge optical elements such as metasurfaces—subwavelength-scale meta-atom arrays that can offer superior characteristics to conventional optics.
The resulting imager has already been put to use by Yao’s team. “My student got a section of a honeybee wing,” Yao recalls. “Looking at it with a conventional microscope, you could see bands and nothing much else, but when we looked at it with the polarization imaging sensor, especially the circular polarization, we found features showing variation in different sections.” If the bees are capable of sensing circularly polarized light, she thinks these polarization features along the wing may help them identify one another.
Having patented the device, Yao believes that with further development in terms of bandwidth, accuracy, scalability, and cost, a polarization-sensitive microscope based on her sensor will be a boon to science and industry.
“There are many applications where circular polarization is going to matter a lot. For instance, detecting the chirality of molecules is very useful for chemistry-related applications, biological applications, and also drug discovery,” she says. “We are not biologists though and we cannot evaluate how significant our discovery is, but I feel like this is probably something very interesting and very new.”
Also developing mantis shrimp-inspired imaging technology are Brendan O’Connor and Michael Kudenov, of North Carolina State University. “Mike and I have been collaborating for a number of years now, looking at how organic photodetectors could be better than other traditional inorganic photodetector technologies for different applications,” says O’Connor.
A researcher holds a chip-integrated polarimetric imaging sensor. Photo credit: Jiawei Zuo/ASU.
Organic photodetectors are optoelectronic devices that convert incident light into electrical signals through the photoelectric effect, utilizing organic materials like carbon-based molecules or polymers as the active absorbing layer. They have the intrinsic advantages of having easily tuneable optical and electric properties, and being simple and cheap to fabricate, making them potentially ideal for numerous sensing scenarios.
For example, they could be used for chemical sensing, mapping pollution locally from factories or globally with drones and satellites. They could also be integrated into wearables to better monitor health metrics, such as blood oxygen, or perhaps skin cancer or certain circulatory diseases. They could even be integrated into smartphones, so that people can measure local air quality, measure meat and grocery quality, and many other functions.
Before Kudenov and O’Connor became aware of the mantis shrimp’s unique visual system, an early invention from their collaboration was an organic polarimeter that actually looked mantis shrimp-inspired, sporting stacked detectors through which different parts of the light’s polarization state could be detected. “And then Mike mentioned the mantis shrimp to me and had the vision to push the technology further to do spectral detection and polarization detection simultaneously, using the mantis shrimp as inspiration,” recalls O’Connor.
The culmination of these efforts is a proof-of-concept sensor that registers four spectral channels and three polarization channels simultaneously, with potential for much more. But it is still very much an academic lab device. To be employed for real-world applications, the sensor will need to be shrunk to a practical size.
“It’s hard to go from a giant cobbled device on a lab bench to a micro-sized, well-packaged detector array; it takes Samsung or similar to be able to fabricate something like that,” says Kudenov, after which O’Connor jokingly adds: “Samsung, if you’re reading this, please follow up if you’re interested.”
Gruev is somewhat closer to commercialization, but for a very specific application. Over the past 10 years, since his first color-polarization sensor, he has devoted significant time and effort to developing mantis-shrimp-inspired sensor technology for cancer imaging, including during cancer surgery.
“When you look at how surgery is performed, it hasn’t changed for 100 years,” Gruev explains. “Before the surgery, there’s all this imaging technology used for diagnostic purposes, but during surgery, I would say that the majority of the time surgeons still use their eyes, touch, and their experience to find the tumor, and to make these critical decisions.”
To assist surgeons and improve outcomes for patients, Gruev and a multidisciplinary team developed color-polarization sensor technology to capture visible light plus near-infrared (NIR) wavelengths, just like mantis shrimp. The sensor consists of three layers of vertically stacked photodetectors covered with a checkerboard-like arrangement of two different filters—one for visible light and the other filtering NIR light. They then integrated the camera in an endoscopic system to be used in solid cancer surgery.
Structures on the wings of honeybees may enable them to sense circularly polarized light. Photo credit: Yu Yao.
Surgery to remove cancer invariably leaves a sliver of cancerous cells behind, which can often lead to the cancer returning. To combat this, fluorescent-guided surgery has been developed, wherein fluorescent tracers are injected into the body to preferentially bind to tumor cells.
The tracers emit a certain wavelength of excitation light that helps surgeons see and remove more cancerous cells. However, tumors can be highly heterogeneous. This means that clinically approved endoscopes that detect one type of tracer will potentially miss different cancer cells that are also present. Pairing Gruev’s single-chip multi-tracer imaging system with appropriate excitation light for activating the tracers has the potential to expand the capabilities of fluorescent-guided surgery and assure doctors and patients of successful results.
Even more recently, Gruev’s team has been extending this technology to the other side of the spectrum: UV. “We are using perovskite nanocrystals to capture UV light and convert it to the visible spectrum, very much like how mantis shrimp actually see UV, to be able to detect positive sentinel lymph nodes,” he says. “If a cancer has spread to other parts of the body, the first place it will have spread will be the lymph nodes. And right now, there’s no optical technology during surgery to detect whether those lymph nodes have cancer or not. Multispectral UV and NIR could be the answer.”
“We’ve been bringing this technology into operating rooms around the world,” Gruev concludes. “Hopefully, we can make a difference.”
Benjamin Skuse is a science and technology writer with a passion for physics and mathematics whose work has appeared in major popular science outlets.