The prodigal microchip
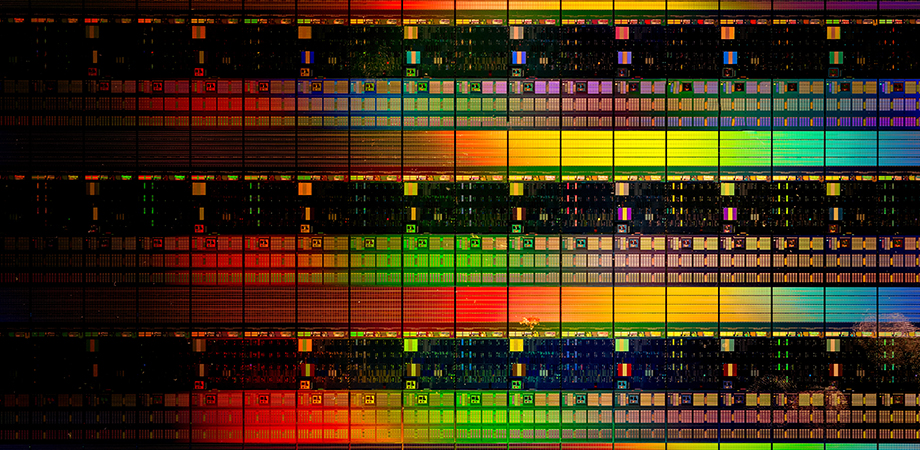
Every microchip is a metropolis.
Despite being a piece of real estate no larger than a fingernail, the modern microchip is home to billions of transistors, miles of metallic interconnects, and layers of structures stacked on top of each other like skyscrapers.
Every second of every day, an endless stream of 1s and 0s move in and out of these transistors riding on the back of zillions of electrons. Like determined travelers, they whiz through metallic highways, halt for signals at the diodes, and wait patiently at the capacitors.
In turn, the controlled chaos of this silicon city runs the world we live in.
They launch ships into space, operate vehicles, do math, physics, and chemistry, and signal the red LED on the electric shaver to blink when the power runs out. One or more microchips runs every one of the 40 billion connected devices currently in use—a figure that's expected to jump to 350 billion by 2030.
As we slowly wake up from the paralysis of 2020, among the many first responders, nurses, doctors, neighborhood shopkeepers, and essential workers to whom we owe an immense debt of gratitude, we should also include the humble microchip. Our ability to cope with the isolation of the past year was made possible through the internet and our connected devices. Every time we hopped on a Zoom call, between our personal devices, routers, data centers, satellites, and peripheral devices, at least a quintillion microchips were called to work.
Unfortunately, like every city, these chips consume an immense amount of resources and generate truckloads of waste.
A "fab" that processes 50,000 wafers—the silicon platform on which chips are built—per month consumes over 1 TWh of electricity a year. That's as much power as is required by a city of 100,000 residents. Moreover, a rough estimate pegs the water consumption of this fab at over 19 million liters per day. That's the amount of water consumed by a city of 60,000—for a whole year!
In addition, these facilities utilize tons of chemicals, most of them expensive and toxic, and generate tons of waste, which include greenhouse gases like SF6, CF4, NF3, and C4F8.
"The electricity that's required to run the fab is the biggest drain on the semiconductor industry," says Sarah Boyd, a sustainability consultant at Sphera. "There are over 1,000 semiconductor fabs operating globally today. They make $450 billion worth of microchips a year and generate 50 million tons of carbon dioxide annually."
These figures are not unknown to the industry. At the 2020 annual meeting of SEMICON West, an important gathering of the semiconductor industry, Gary Dickerson, CEO of Applied Materials, stated that his company generates 145,000 metric tons of CO2 a year. For comparison, the global electronics industry as a whole generates a billion tons of CO2 annually.
Given the size of the microchip, these numbers seem extraordinary. However, this could very well be the price that we pay for the complexity of a chip, and the comfort it brings into our lives.
The microchip is essentially made from sand—albeit sand that has been melted, purified, and refined until it is over 99.9999 percent pure silicon.
The arduous task of turning these disc-shaped, purple-colored wafers into microchips and memory devices fall on the fabs, which are high-tech facilities scattered across the world, with the majority in Southeast Asia.
The elements of lithography, sand and silicon crystals, sit atop a silicon wafer. Credit: ASML
Chip manufacturing is a complex process. Several billion transistors, electrical contacts, capacitor devices, and other peripherals need to be created on a silicon wafer. Some parts of the transistor need to be implanted with elements that donate an electron, like phosphorus, and other parts need to be implanted with elements that absorb an electron, like boron. Expensive and precious metals need to be laid down on precise locations, at the correct thickness. With a typical transistor being no larger than a few nanometers across, all of this has to be highly accurate and scalable.
The solution is to carve a microchip layer by layer using light, in a process called photolithography.
First, the whole wafer is coated with a polymer called a photoresist that has the property of hardening when exposed to light. Ultraviolet rays are shone on the wafer through a special mask that has been selectively patterned with holes. The parts of the wafer in the shadow of the mask are unexposed to UV rays and are easily washed away by a solvent. These regions are coated with a fresh layer of metal, or more dielectrics, until they are filled up.
Now the whole process repeats for the second layer—and the one after that. Each layer is cleaned with chemicals after UV exposure and then heat treated. The tiniest impurity can drastically alter the property of silicon and make transistors useless. Therefore, the wafers need to be cleaned repeatedly with a pristine form of water that is very expensive to produce.
The stringent requirements on purity and cleanliness require that fabs turn themselves into unusually sterile environments. This necessitates a complex ecosystem of air conditioners, water purifiers, vacuum pumps, cryogenic systems, lasers, plasma chambers, and chemical circulation systems that operate on a continuous loop, as long as the fab is running.
This complex semiconductor fabrication process is nestled at the heart of an elaborate web of international assembly lines. The company that makes the wafers and the fabs that create the microchips can be located in different parts of the globe. The assembly of the actual device likely takes place in a different company at a third location, and the end user could be anywhere in the world.
This means that the company whose name is on the final product might have very little control over the conditions and practices of the fabs. Further, different parts of the semiconductor lifecycle are regulated by different environmental legislations, making not just the implementation of sustainability efforts, but also the tracking of their environmental footprints, complicated.
"The environmental footprint of the semiconductor industry is not easy to determine, because the industry lacks a holistic approach to assess the impact of chip manufacturing accurately. While manufacturers do try to reduce their environmental footprints, the full material and energy flow across the whole system is usually unavailable," says Marie Garcia Bardon, senior researcher at IMEC.
Boyd agrees. "The main challenge in determining the environmental footprint of a chip is access to data, as the exact energy, water, and chemical requirements of a process are commercially sensitive and closely guarded by fabs," she says.
Yet, the lithography process is where we could save the most energy and resources. A 2002 study stated that only 20 percent of the total energy consumed by a car was attributable to the original manufacturer—and the stats haven't changed much in 20 years. In contrast, manufacturing accounts for 50 percent of the total energy consumed over the lifespan of an average electronic device. So, in order to reduce the environmental impact of a car, we could make driving more efficient; but in order to reduce the impact of a microchip, we have to manufacture them more efficiently.
The EUV source vessel inside ASML's NXE3400 EUV machine. Credit: ASML
Dean Freeman, chief analyst at Freeman Technology and Market Advisors, agrees. "Reducing the amount of water that is used to clean wafers in the fab and removing contaminants requires us to innovate our manufacturing process. It will require new types of lasers, plasma processes, and new photoresists that do not contain metals." He says these are active areas of research.
ASML has been at the forefront of the extreme ultraviolet lithography (EUVL) process—a technique used to create transistors in sub-10 nm dimensions. Samsung, for example, has fully integrated EUVL into its manufacturing process and provides these new chips to many industry leaders such as Apple, Qualcomm, and IBM.
Freeman calls EUVL "one of the ten biggest miracles of the modern world." Indeed, the EUVL process is extremely complicated—it produces very high-energy ultraviolet rays by bombarding vaporized tin atoms with a carbon dioxide laser, and then steering these rays to the wafer surface using an array of highly reflective lenses. The development of this process began over two decades ago and has involved active collaboration from a broad section of the industry.
Marijn Vervoorn, director of sustainability strategy at ASML, points out the improved impact of this process on sustainability goals. "EUV lithography is a critical scaling engine that enables more energy-efficient data centers and devices, reducing the overall footprint of use. EUV lithography enables more efficient production of microchips and devices by reducing the number of production steps required and making equipment as energy efficient as possible."
Indeed, as transistors get smaller, they get closer to each other within the microchip. This reduces the power required to switch them, as well as the energy lost in moving electrons.
"This effect is the rule of scaling," Garcia Bardon observes. "However, we do tend to use more microchips as time goes on," meaning that whatever energy we save by reducing the size of transistors could be easily lost by the larger number of chips that we use. Boyd agrees. "The induction effect is when as chips get smaller, they get cheaper," she explains. "But then the rebound effect kicks in, meaning that as a society we use more of them globally." So, it is unclear whether EUVL represents the sort of process innovation that leads to a better environmental footprint for the semiconductor industry. The same holds true for many of the advanced lithography techniques being developed for the next generation of smaller transistors, such as maskless lithography and nanoimprint lithography.
But what we do know is that there are fundamental thermodynamic limits to how much energy we could save. For example, it does take a certain amount of energy to cleave silicon from the silicon dioxide in sand and purify it. We could make that process more efficient so that less heat is wasted, but we could not go below the minimum energy dictated by chemical thermodynamics.
Overall, a microchip is a structure that stands in abject defiance of the second law of thermodynamics: It creates a region of extreme order from a whole lot of chaos, and that does require a lot of energy.
"The most effective way to reduce the carbon footprint of chips is to ensure that the energy consumed by a fab is generated using renewable sources," points out Boyd. "The use of renewable energy is where we could see the most impact of sustainability efforts."
Indeed, many industry leaders already place renewables high on their sustainability roadmap. In his keynote, Dickerson announced that Applied Materials is on track to source all its energy requirements from renewables in the US by 2022, and worldwide by 2030. ASML, one of the leading providers of lithography equipment to fabs around the world, fully runs on renewable energy. The Taiwan Semiconductor Manufacturing Company (TSMC), one of the largest fabs in the world, has announced their commitment to 100 percent renewable energy and zero indirect carbon emissions from electricity consumption by 2050. Intel purchases enough renewable energy to cover more than 70 percent of its electricity use worldwide. They plan to be based on 100 percent renewable energy by 2030.
And, although fabs use enormous amounts of water in chip production, they also recycle and reuse much of the water that runs into their wafers. Boyd's model calculates that there has been anywhere from a two to ten-fold reduction in water use for semiconductors if you consider the water that's being recycled and reused. "There have certainly been major improvements since the 1990s," she says.
Even with these developments, it is likely that microchip manufacturing will continue to be a major consumer of electricity, water, and chemicals. We could ensure that the energy is supplied by renewables, that the water is recycled, and the chemicals are processed without damage to the environment. Yet, the total amount of these resources that are demanded by the industry, and the lithography process specifically, is likely to be quite high.
Unfortunately, this puts semiconductors in direct competition with other resource-intensive industries such as agriculture, or even the local community, in locations such as New Mexico (the site of an Intel fabrication facility), where water is a scarce resource.
However, semiconductor manufacturing appears cognizant of its role in moving towards a more sustainable future.
"Among industries, the semiconductor industry has the potential to be more sustainable. Just a standard fab is usually operated quite environmentally responsibly," adds Boyd. "The issue at the policy level is to incentivize more sustainability efforts."
In other words, we must be relentless in our efforts to make microchips more sustainable. And we should never forget that the comforts of modern life gifted by these wonder chips come at the expense of a vast amount of resources.
Vineeth Venugopal is a scientist and science writer who loves all things and their stories.
Enjoy this article? Get similar news in your inbox |
|