Healthcare's wearable future
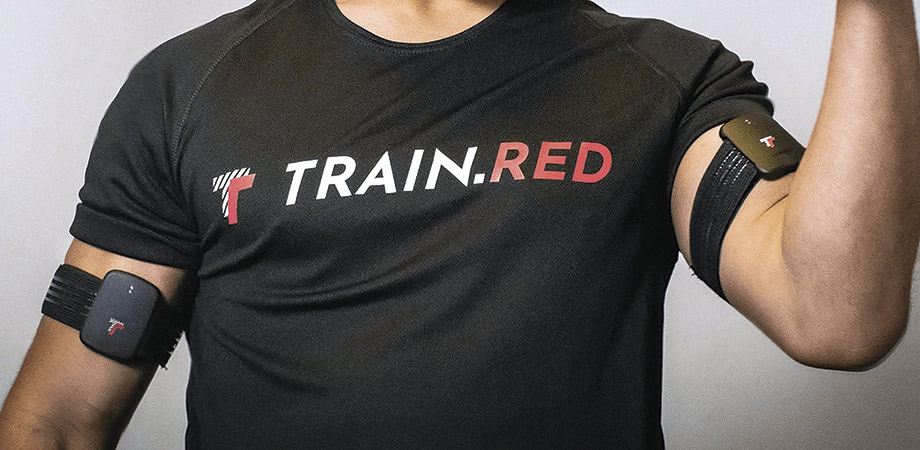
As you step out of your house into Monday morning sunshine, a heart-shaped patch on the back of your hand turns purple. There is more UV light today than usual—you run back inside to put on some sunscreen lotion.
All that activity has elevated your heart rate, and your smartwatch is blinking furiously, judging whether this is routine cardiovascular exercise or sudden-onset arrhythmia. The watch checks with a sensor placed discreetly over your chest and confirms that there is no cause for worry.
Yet another sensor confirms that your blood glucose and creatine are within normal limits, though the alcohol level is still minimally elevated from a weekend wedding party. Two separate sensors placed over the calf muscles report lower than usual metabolism, which might be due to the statins you took in the morning. Whatever the cause, the sensors agree: It’s time for you to go in for a checkup.
Thanks to a plethora of photonic devices that have become proficient at reading the human body, this scenario is not far from reality.
Whether as sensors of blood oxygen, heart rate, alcohol, glucose, or one of the ever-growing lists of additional human-health biomarkers to monitor, wearables are becoming an inherent part of our daily lives.
“Twenty percent of people have wearable devices,” says Bruce Tromberg, director of the National Institute of Biomedical Imaging and Bioengineering (NIBIB), which is part of the US National Institutes of Health.
These wearables are the beginning of a profound shift in healthcare systems that enable preventive medicine instead of the current paradigm of corrective treatment. Not only does preventative medicine save trillions of dollars in annual healthcare costs, but it will also lead to a healthier, more empowered citizenry.
This was particularly evident during the worst months of the coronavirus pandemic when wearable electronics were one of the few market segments to grow.
The market-research agency Gartner estimates that worldwide spending on smartwatches increased from $18.5 billion in 2018 to $31.3 billion in 2022. These devices not only helped an angsty population strive for better health, but also helped them self-monitor for covid symptoms at a time when access to medical care was often difficult.
“Apple watches were used as a surrogate for oxygen sensors [during the pandemic],” says Tromberg.
Wearables, however, are on the cusp of a transformation. Driven by photonics and increasingly aided by microelectronics, machine learning, and artificial intelligence, wearable devices find application across a broad spectrum of human biology, from measuring neurological functioning to musculoskeletal health. They are being used by everyone from children to aging adults, and from the average office worker to high-
performance athletes.
Though previously viewed as flashy devices and status symbols, they are helping create a “data ecosystem” of the human body, allowing unprecedented real-time monitoring of all aspects of our physiology. By combining sensors for various biomarkers, and by placing them at several locations around the body, we are able to create a real-time map of our biochemistry and see how that relates to things like athletic performance, physical endurance, muscle failure, and recovery.
A data ecosystem enabled by wearables could eventually be highly disruptive to the healthcare industry, which according to Tromberg, “takes up around 30 percent of US GDP.”
Aaron Zilkie calls it the “mapping of the human biome”—an effort to gather a large set of measurements capturing the physiology happening inside us at any given moment—an endeavor that is no less daunting than the mapping of the human genome.
Zilkie is the chief technical officer of Rockley Photonics, which makes miniaturized spectrometers to track a wide range of substances in the blood, including water, alcohol, lactose, urea, and glucose, in addition to monitoring body temperature, blood pressure, and heart rate, all with a matchbox-sized device worn on the wrist.
Other scientists, like Conor Evans at Massachusetts General Hospital, use a multiplicity of sensors to track the relationships between localized changes in the body—say, around the arm, back, or chest—and systemic changes such as an increase in body temperature.
“Having multiple sensors allows you to better infer what’s going on both locally and globally,” he says.
At the University of Georgia, Kevin McCully measures athletes’
muscle oxygenation using an off-the-shelf near-infrared (IR) spectrometer. In exercise physiology, measurements of such systemic changes like oxygen consumption or metabolism can be used to track and guide exercise intensity and physical conditioning.
Muscle oxygenation can be measured with an off-the-shelf near infrared spectrometer. Photo credit: Kevin McCully
McCully, who directs University of Georgia’s Exercise Muscle Physiology Laboratory, first measures the rate of oxygen consumption in a resting muscle. He repeats the process after cutting off blood supply with a blood pressure cuff. Next, the spectrometer beams near-IR
radiation into the skin. Hemoglobin, the iron-containing oxygen transport molecule that gives blood its bright red color, strongly absorbs at 850 nm and, when deoxygenated, at 760 nm. The difference in reflected radiation wavelengths between the nonconstricted and constricted states is a measure of the muscle’s metabolism.
McCully next measures the change in muscle metabolism between states of rest and exercise, which informs us about the energy production in the tissue. “Your muscles may increase oxygen consumption by fifty-fold when you exercise,” he says.
These readily available and wearable near-IR sensors can have an enormous impact not just on athletic performance, but on an athlete’s overall health. “Twenty percent of speed skaters have some disease of the arteries from their training,” McCully says. Carefully measuring the oxygen intake of muscles on and off the rink can help in making subtle adjustments to their training routines, such as changing the angle of the knee slightly during skating, which in turn can lead to improved performance and shorter recovery.
It’s not just speed skaters and other high-level athletes who benefit from these sensors, however. People who suffer from muscle aches as a side effect of prescription drugs might want to get their muscles evaluated, and so too, the elderly. “Older adults tend to have poor circulation in their legs, particularly if they’re diabetic or if they smoke,” says McCully.
Near-IR light penetrates the skin to just below the
epidermis. “This wavelength range includes the skin’s so-called water windows region of transparency to optical radiation,” says Evans. These measurements assume that the oxygen availability in the blood is representative of the tissue that it serves. And this need not be the case all the time, especially if the tissue is damaged.
He has been pioneering a new modality for oxygen sensing using so-called smart bandages. These are worn on the skin just like regular bandages. They contain a chemical called porphyrin that changes color in response to low levels of oxygen, a condition also known as hypoxia.
Evans and his colleagues developed a stronger type of porphyrin for use as a sensor in the bandages. The chemical is so deeply emissive that a few nanograms can be seen under a penlight. The emission of this porphyrin is suppressed by oxygen, which is why the color is brightest at low concentrations.
On the skin, the bandage can measure an aggregate oxygen level from the molecular oxygen seeping through tissues as well as from other sources such as sweat. That’s because, if the oxygen content in a tissue is falling, it will bring the average down all around it, causing the smart bandage to change color. This visible color change can be integrated with digital electronics for remote monitoring by a doctor, for example.
Bandages with a chemical sensor are low-cost and easy to manufacture. Aydogan Ozcan at the University of California, Los Angeles, has developed paper-based point-of-care (POC) multiplexed sensors that can perform 100 immunoreactions within 15 to 20 minutes. The devices can quantify a large panel of biomarkers with a high degree of precision.
“Think of this as 100 tests, performed all in parallel, using a paper-based disposable POC sensor,” he says. The device costs less than a dollar and produces a photonic signal that can be read using a smartphone. It can detect Lyme disease in addition to monitoring cardiovascular function.
A paper-based disposable sensor that detects Lyme disease. Photo credit: Aydogan Ozcan
As wearables become more widespread, the ability to measure more than one human-health biomarker on just one device becomes significant. Most people would not want to be bedecked with a bunch of different smart devices for measuring different biomarkers.
Rockley Photonics uses integrated silicon photonics for its “clinic on the wrist.” Using more than 100 different lasers, each with a diameter of only a few micrometers, the company has created a spectrometric device that scans in a wide range of wavelengths, including the visible and near IR. Since a particular type of laser only generates light of a fixed wavelength, the Rockley system combines many different types of lasers in a Lego-like fashion on a silicon chip to capture a broad range of radiation.
Radiation emitted by the device reaches into the capillaries and fluids at the edge of tissues, providing a real-time assessment of several biomarkers.
“Brain scanning is something we’d like to do,” Zilkie says. “By mapping the 3D oxygen flow in the brain, one can determine what the brain is doing.” This could one day lead to better measurement and evaluation of our real-time cognitive skills.
Photonics-driven wearables might also spur progress in studies of sleep. “We know very little about sleep,” says Tromberg. “It’s an easier measurement as there is no motion artifact.” He means that wearable devices that read sleep signals do not need to adjust for other body movements and external noise. Multiple sensors placed at different parts of the body could aid understanding of not only how we sleep, but also why we sleep.
By consistently tracking vital functions through a large set of biomarkers, we can assess risk factors quickly and far more efficiently than we do today. Data from wearables can help categorize people into different risk categories that can then be used to decide who needs to go for a more expensive diagnostic exam like magnetic resonance imaging (MRI).
Tromberg labels imaging technologies like MRI as “big physics,” which are only available in healthcare settings and are hard to miniaturize. Leveraging insights from wearables “can lead to big physics sooner,” he says. It also makes sure that we are doing big physics at the time when it is most useful in a patient’s diagnosis. This can significantly cut down costs of tertiary healthcare, the extremely specialized care that we receive in hospitals using complex advanced equipment, procedures, and highly trained personnel.
As wearables develop further as diagnostic tools, we might be able to detect sooner tell-tale signs of neurodegenerative diseases like Alzheimer’s and Parkinson’s. These new, portable diagnostic tools might also lead to a significant reduction in healthcare costs, particularly for the elderly.
Yet another way that wearables can disrupt healthcare is by reducing reliance on trained personnel through machine learning-based augmentation of data analysis. In the future, an artificial intelligence algorithm that monitors the signals from your wearable ecosystem could tell you when it is time to schedule a checkup or have your liver function tested. This can be especially useful in high-stress environments and remote locations such as the frontlines of war zones, where both personnel and time can be in short supply. The AI system could suggest what medicines to take, which procedures to perform, or who gets priority for surgery—tasks that are now typically performed by humans.
Artificial intelligence can take this even further. Aydogan’s team has developed a passive diffractive neural network, which is layers of materials stacked together in such a way that light propagates in a very specific manner through them. These networks process light in the same way that a powerful computer would do and hence can provide far more information than wearables by themselves.
“Such diffractive all-optical processors provide unmatched opportunities. They complete their signal processing tasks in literal picoseconds as the light travels through materials as thin as a stamp, and they do not consume power during these computational tasks,” he says.
In March 2022, the Biden-Harris Administration announced the “Test to Treat” initiative for covid-19, which aims to help people quickly access lifesaving treatments for covid at facilities that integrate testing, clinical insights, and medical prescriptions. Wearables extend this idea to all aspects of healthcare by enabling better metrology and insights into our own health.
As more and more sensors continue to poke us with needles of light, a hopeful future awaits just beyond the horizon, illuminating us from within.
Vineeth Venugopal is a science writer and materials researcher who loves all things and their stories.
Learn about the latest research on wearables at Photonics West.